Vascular Biology Lab – Shear
Stress
Haemodynamic forces are important modulators of vascular tone and vascular wall remodelling, and are increasingly implicated in many physiological and pathological functions. Blood vessels are under the influence of two primary haemodynamic forces: The circumferential force - the wall tension by the blood pressure and, secondly, the frictional force or shear stress which results from blood flow along the vessel wall. The shear stress experienced by the endothelium is a function of the ‘axial’ pressure, which occurs as blood flows through the vessel and, physiologically, is of the order of 0–50 dyn/cm2. Shear stress could be transduced into a wide range of associated intracellular biochemical events. Blood flow was first recognized to be important in the control of vascular tone in 1933 (Schretzenmayr, 1933), in femoral artery of dog. It is now recognized that the endothelial cell, uniquely situated at the interface between the blood and the vascular wall, is effectively a biological mechanotransducer which senses shear forces and converts these physical stimuli to intracellular biochemical signals.
Shear stress appears to be a particularly important hemodynamic force because it stimulates the release of vasoactive substances and changes gene expression, cell metabolism, and cell morphology. The nature and magnitude of shear stress plays an important role in long-term maintenance of the structure and function of the blood vessel. While steady shear stress generally stimulates many of the same endothelial cell responses as pulsatile stress, oscillatory shear stress leads to pathological conditions like atherosclerosis, through endothelial damage. Areas of low shear stress may be more prone to intimal lesion formation, because in these areas the endothelium may produce less vasoprotective factors, such as NO and prostacyclin unlike normal shear stress. Considering the beneficial effects of NO and prostacyclin, it could be speculated that the spatial distribution of atherosclerotic plaques in areas of low shear stress may in part be explained by a reduced elaboration of NO (decrease in eNOS) and prostacyclin at these sites. This reduction in NO levels leads to decrease in the endothelial functions.
Cardiovascular diseases, particularly ischemic heart disease and myocardial infarction, are the major concerns presenting a major scientific challenge for the development of optimal therapies.The mechanosensors on the endothelium that sense changes in blood flow and shear stress are poorly defined at the molecular level. Our lab focuses on shear stress mediated regulation of functional and mechanistic aspects of endothelium and blood vessels.
PHYSICS OF SHEAR STRESS
STRAIN
Strain is the
geometrical expression of deformation caused by the action of stress
on a physical body. Strain therefore expresses itself as a change
in size and/or shape. When a body is subjected to a load (force),
it is distorted or deformed. If the load is small, the distortion
will probably disappear when the load is removed. Such a proportional
dimensional change-intensity, or degree, of distortion is known
as strain.
If strain is equal over all parts
of a body, it is referred to as homogeneous strain; otherwise, it
is inhomogeneous strain. Strain,
is given by

where:
e = strain (in./in.)
d = total elongation (in.)
L = original length (in.)
Elastic
strain is a transitory dimensional change that exists only
while the initiating stress is applied and disappears immediately
upon removal of the stress.
Plastic strain
is a dimensional change that does not disappear when the initiating
stress is removed.
STRESS
Stress
is the internal resistance, or counterforce, of a material to the
distorting effects of an external force or load. These counterforces
tend to return the atoms to their normal positions. The total resistance
developed is equal to the external load. This resistance is known
as stress.
Stress (s) can be equated to the
load per unit area or the force (F) applied per cross-sectional
area (A) perpendicular to the force. The external load and the area
to which it is applied can be measured by the equation below:
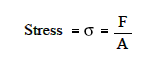
Where:
s = stress (psi or lbs of force
per in. 2)
F = applied force (lbs of force)
A = cross-sectional area (in. 2)
TYPES OF STRESS
Stresses occur in any material
that is subject to a load or any applied force. There are many types
of stresses, but they can all be generally classified in one of
six categories: residual stresses, structural stresses, pressure
stresses, flow stresses, thermal stresses, and fatigue stresses.
Fatigue stresses
are due to cyclic application of a stress. The stresses could be
due to vibration or thermal cycling. When loadings are cyclic or
unsteady, stresses can effect a material more severely. Stress intensity
within the body of a component is expressed as one of three basic
types of internal load. They are known as tensile, compressive,
and shear.
Mathematically, there are only two
types of internal load because tensile and compressive stress may
be regarded as the positive and negative versions of the same type
of normal loading. This is illustrated in the following diagram:
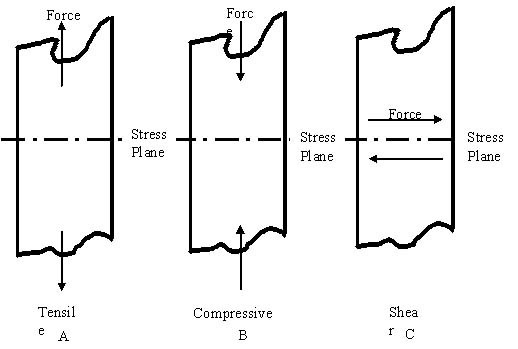
Tensile
stress is that type of stress in which the two sections
of material on either side of a stress plane tend to pull apart
or elongate as illustrated in figure (A).
Compressive stress
is the reverse of tensile stress. Adjacent parts of the material
tend to press against each other through a typical stress plane
as illustrated in figure (B).
Shear stress
exists when two parts of a material tend to slide across each other
in any typical plane of shear upon application of force parallel
to that plane as illustrated in figure (C).
While the plane of a tensile or
compressive stress lies perpendicular to the axis of operation of
the force from which it originates, the plane of a shear stress
lies in the plane of the force system from which it originates (depicted
as below).
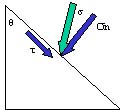 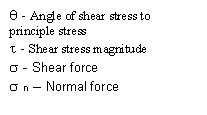
Two types of stress can be present
simultaneously in one plane, provided that one of the stresses is
shear stress. Under certain conditions, different basic stress type
combinations may be simultaneously present in the material. An example
would be a reactor vessel during operation. The wall has tensile
stress at various locations due to the temperature and pressure
of the fluid acting on the wall. Compressive stress is applied from
the outside at other locations on the wall due to outside pressure,
temperature, and constriction of the supports associated with the
vessel. In this situation, the tensile and compressive stresses
are considered principal stresses. If present, shear stress will
act at a 45° angle to the principal stress.
SS-
Pathology
-
Implication
-
Cardiovascular blood flow
-
Atherosclerosis
-
Portal hypertension
Implication
Cells in situ are always subjected
to varied mechanical stresses including gravitational force, mechanical
stretch or strain, and shear stress. Especially the endothelial
cells (ECs) in particular, which form the inner lining of the blood
vessels are constantly exposed to hemodynamic forces in the form
of shear stress due to the pulsatile nature of blood pressure and
flow. Endothelial cells also respond differently to different modes
of shear forces, e.g., laminar, disturbed, or oscillatory flows.
ECs lining the vessel wall recognize
shear stresses convert mechanical stimuli into intracellular signals
that affect cellular functions like proliferation, migration, permeability,
cytoskeleton remodeling, and gene expression as well. ECs transduce
signals to vascular muscular cells and others in order to modify
vessel shape and structure accordingly.
How do cells, and the endothelium
in particular, sense a change in shear stress, and what are the
signaling pathways for the cellular response? From a simplistic
standpoint, changes in fluid shear stress could be sensed directly
by cell membrane components such as membrane proteins, ion channels,
or caveolae or by alterations of the cellular cytoskeleton; subsequent
cellular signaling cascades through phosphorylation events or generation
of reactive oxygen species (ROS) can lead to diverse effects such
as the release of cytokines and other mediators, activation of transcription
factors, altered gene and protein expression, and cell division
or death.
Cell Response to
shear stress
In vitro studies on cultured ECs
in flow channels have been conducted to investigate the molecular
mechanisms by which cells convert the mechanical input into biochemical
events, which eventually lead to functional responses. The knowledge
gained on mechano-transduction, with verifications under in vivo
conditions, will advance our understanding of the physiological
and pathological processes in vascular remodeling and adaptation
in health and disease.
Cardiovascular
blood flow
Different forms of heart disease
can be caused by atherosclerosis (hardening or furring of the arteries).
Atherosclerosis is caused by the formation of plaques that bulge
into the artery, narrowing the blood vessel. Cardiovascular diseases,
particularly ischemic heart disease and myocardial infarction, are
the major concerns presenting a major scientific challenge for the
development of optimal therapies.
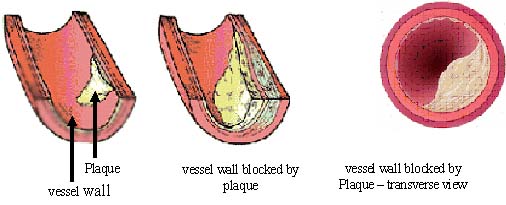
Cerebral strokes, another vascular
disorder, are another points of concern. These vascular diseases
share a common factor - atherosclerosis and the failure or destruction
of the vascular wall structure. This mechanical disruption of the
vascular wall specifically the arterial wall, leads to fatal outcomes
such as acute coronary syndrome and sub-arachnoid hemorrhage. Therefore,
both the onset and final consequences of tragic fatal vascular diseases
are inseparably connected to mechanical events that occur on the
vascular wall, which are likely influenced by alterations in blood
flow.
Atherosclerosis
Atherosclerosis is an inflammatory
process disease that involves the artery wall and is characterized
by the progressive accumulation of lipids, cholesterol, calcium,
and cellular debris within the intima of the vessel wall, endothelial
dysfunction and vascular inflammation. This buildup results in plaque
formation, vascular remodeling, acute and chronic luminal obstruction,
abnormalities of blood flow, and diminished oxygen supply to target
organs. Atherosclerosis preferentially occurs at specific arterial
sites, branches, bifurcations and bends, and this phenomenon is
thought to be related to hemodynamics.
One factor that determines how
easily an atherosclerotic plaque forms is the wall shear stress
that the blood flow puts on the walls of the blood vessels. Under
normal shear stress a normal production of Nitric oxide, prostacyclin,
thrombomodulin and Plasminogen activator is evident, which acts
as Anti-thrombic agents, while NO and TGF-ß are anti Atherosclerotic.
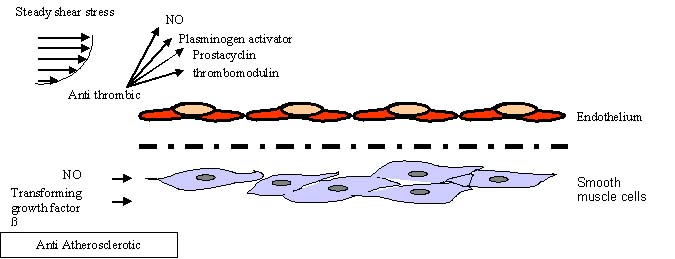
With low shear stress (LSS) and
oscillatory shear stress ( OSS) being pro-atherogenic. It was shown
that LSS induces the production of more IP-10, GRO-alpha (chemotactic
factors) and fractalkine (membrane-bound soluble protein) than OSS,
which in fact induced no fractalkine. This correlated with differences
in the composition of the atherosclerotic plaques: LSS induced plaques
with thinner fibrous caps and larger necrotic cores than OSS, which
is associated with plaque rupture that can cause heart attacks.
Low shear stress is associated
with increased wall thickening and accelerated lumen narrowing in
moderately diseased human coronary arteries. There is a continuous
inverse relation between shear stress and rate of lumen narrowing.
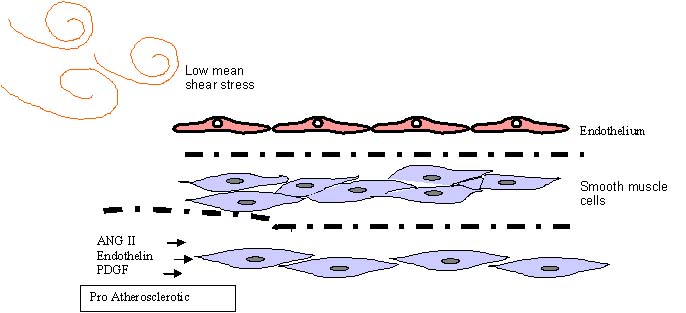
Areas of low shear
stress may be more prone to intimal lesion formation, because in
these areas the endothelium may produce less vasoprotective factors,
such as NO and prostacyclin unlike normal shear stress. Considering
the beneficial effects of NO and prostacyclin, it could be speculated
that the spatial distribution of atherosclerotic plaques in areas
of low shear stress may in part be explained by a reduced elaboration
of NO (decrease in eNOS) and prostacyclin at these sites. This reduction
in NO levels leads to decrease in the endothelial functions.
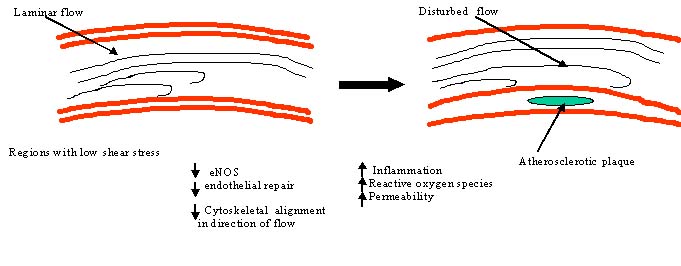
While low shear stress is associated
with atherogenesis and disease progression, regions of moderate
to high shear stress are relatively spared of intimal thickening
as long as flow remains unidirectional and axially aligned.
Increase in endothelial shear
stress (due to increase in flow) is associated with enlargement
of non-atherosclerotic arteries, as seen in arteriovenous fistulas.
The same phenomenon may occur in atherosclerosis. As plaque impinges
on the vessel lumen, endothelial shear stress increases. This might
be expected to trigger the same remodeling response that occurs
in other settings of increased shear stress.
Portal
hypertension
Portal hypertension is defined by
an elevation in blood pressure in the portal system. Either an increase
in blood flow, an increase in resistance, or both elevates portal
pressure. In the normal liver, intrahepatic resistance changes with
variations in portal blood flow, thereby keeping portal pressure
within normal limits. In cirrhosis, however, both intrahepatic resistance
and splanchnic blood flow are increased. Normally, blood is carried
to the liver by a major blood vessel called the portal vein. If
blood can’t flow easily through the liver because of cirrhosis,
the blood gets slowed down in this vein and the pressure inside
the vein increases. This higher blood pressure in the portal vein
is called portal hypertension.
If blood can’t flow normally
through the portal vein, it must return to the heart using other
blood vessels. These vessels become swollen, called Varices because
of the increased amount of blood flowing through them. Varices have
thin walls and can easily break open because they aren’t meant
to handle such high-pressure blood flow, which can be very fatal.
It is firmly established fact that
NO production is stimulated by shear stress and shear stress and
portal hypertension are related. In chronic liver injury, the molecular
basis of the intrahepatic NO deficiency has uniformly been attributed
to a decreased activity of the endothelial isoform (eNOS). Based
on organ-specific distribution of NOS, there could be an important
aspect of the role of NO in portal hypertension. Besides a decreased
production, there might also be an increased degradation of NO because
of enhanced superoxide activity as a result of superoxide dismutase
deficiency, leading to diminished bioavailability of NO.
Modulating the hepatic system by
decreased intrahepatic vascular resistance (IHVR) while maintaining
or enhancing hepatic blood flow. Furthermore, the vasodilatory effect
should be limited to the hepatic microcirculation to prevent a worsening
in splanchnic/systemic vasodilatation.
Recent works suggest that shear
stress is a biophysical stimulator for nitric oxide (NO) production,
which is known to be involved in a wide range of physiological as
well as pathophysiological developments including vascular remodeling.
Animal, molecular, and cellular studies of the endothelium's response
to hemodynamic shear stress have provided new insights into the
possible link between shear stress and cellular migration. Cellular
migration is highly implicated in vascular remodeling and angiogenesis.
Although published data clearly show that shear stress is determinant
of both NO production and migration in endothelial cells (EC) the
inter-relationship between SS, NO and EC migration is not yet known.
Our aim is to unravel the mechanisms by
which SS mediated migration of EC is coupled with the NO generation.
Techniques:
Our approaches are mainly based
on cell and molecular biology techniques using Shear Stress Apparatus,
live cell migration detection by Boyden’s chamber, live cell
chamber and atomic force microscope (AFM) and gene and pharmacological
delivery of NO.
Shear
stress Apparatus
The
cells used in this study are endothelial cells. Endothelial cells
form a multi-functional lining of the intimal surface of blood vessels.
This lining is continuously subjected to both steady and oscillatory
fluid shear stresses, resulting from the flow
of blood in the circulation.
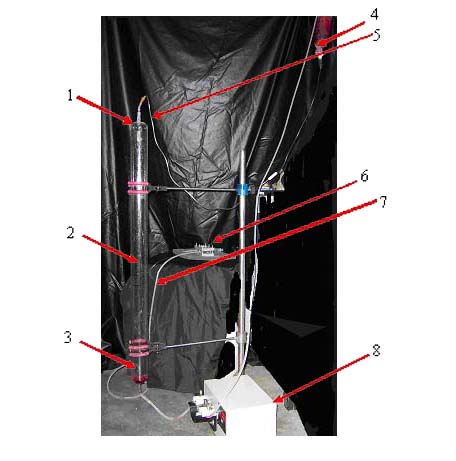 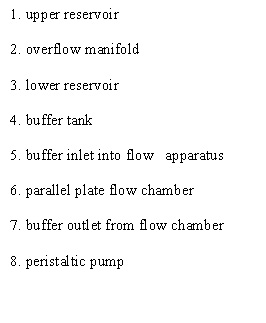
Shear stress consists of two parts
– Parallel plate flow chamber and Flow apparatus. The flow
chamber, a polycarbonate plate, a rectangular Teflon gasket, and
the glass slide with the attached endothelial cell monolayer. (We
are thankful to Dr. Vijay Shah, Mayo Clinic, Rochester for providing
the Parallel plate apparatus)
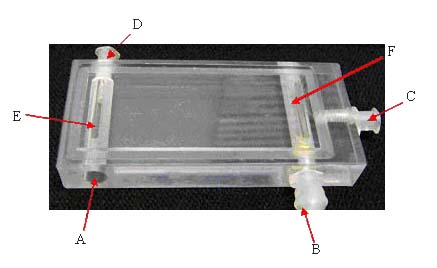
Parallel plate flow
chamber assembly: the plate holder holds Polycarbonate plate (1
below), gasket and the glass slide with the cells attached on it,
together (2-3 below). The medium enters through the entry port (A),
through the slit (E), into the channel, and exits through the slit
(F), and exit port (B). Valve (D) serves to remove the bubbles entered
through the entry port (A).
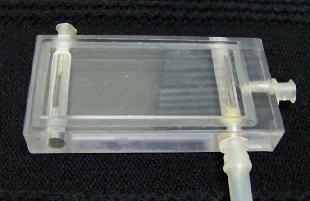 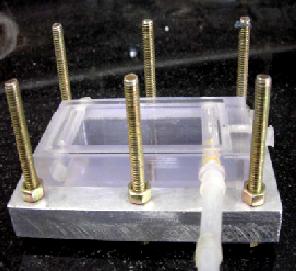 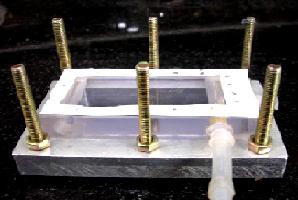 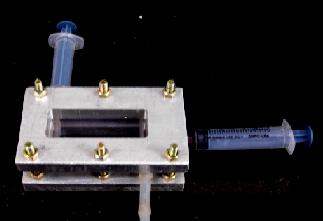
A flow apparatus is capable of
subjecting cultured cells to a wide range of steady shear stresses
for long time periods. The apparatus consists of two reservoirs,
situated one above the other, with a parallel-plate flow chamber
positioned between them. The flow chamber is held in a metallic
chamber to eradicate any leakage of the medium from it. Flow is
driven through the chamber by the hydrostatic pressure head created
by the vertical distance between the upper and lower reservoirs.
The pressure head is maintained constant by continuous pumping of
culture medium from the lower to upper reservoirs at rates in excess
of that flowing through the chamber. The excess drains down the
glass overflow manifold, which also serves to facilitate gas exchange
with the medium. The reservoirs are made of glass, while the interconnecting
tubing consists of Tylon except for the section through the roller
pump, which is silicone. Silicone collars join the reservoirs to
the manifold and tubing. The relatively inert and gas impermeable
Tylon tubing prevents water and gas loss and minimizes absorption
of cell metabolites.
Calculations:
The wall shear stress on the cell
monolayer in the flow chamber may be calculated using the formula:
t = 6Qm/bh 2
where Q - flow rate (cm 3 /s);
m - viscosity (ca. 0.01 dyn s/cm
2)
h - channel height (0.019 cm)
b - slit width (2.1 cm)
t - wall shear stress (dyn/cm
2 ).
Table for different
ranges of shear stress and corresponding height of the parallel
flow plate:
Sl.
No |
Shear
stress (dynes/ cm 2) |
Height
(in cms) at which the flow chamber placed (from the ground
level) |
1
|
1
|
110
|
2
|
5
|
98
|
3
|
10
|
75
|
4
|
15
|
57
|
5
|
20
|
39
|
6
|
25
|
18
|
References
Oren Traub; ; Bradford C. Berk.
Laminar Shear Stress Mechanisms by Which Endothelial Cells Transduce
an Atheroprotective Force. Arteriosclerosis, Thrombosis, and Vascular
Biology. 1998.18:677-685.
Kristopher S Cunningham and Avrum
I Gotlieb. The role of shear stress in the pathogenesis of atherosclerosis.
Laboratory Investigation 2005. 85: 9–23.
Song Li, Ngan F. Huang, and Steven
Hsu. Mechanotransduction in Endothelial Cell Migration. Journal
of Cellular Biochemistry. 2005 96:1110–1126.
Aron B. Fisher, Shu Chien, Abdul
I. Barakat, and Robert M. Nerem Endothelial cellular response to
altered shear stress. Am J Physiol Lung Cell Mol Physiol. 2001.
281: L529-L533.
Mitchell.D.Botney. Role of Hemodynamics
in Pulmonary Vascular Remodeling Implications for Primary Pulmonary
Hypertension. Am J Respir Crit Care Med 1999 Vol 159. pp 361–364.
Research Labs working on Shear stress
Song Li
Group
http://ctelab.berkeley.edu/research/mechanotransduction.htm
Prof. Morton
Friedman
http://www.bme.duke.edu/faculty/friedman/index.php
Konstantinos
Konstantopoulos
http://engineering.jhu.edu/~cheme/fp/e/?id=182
Schwartz
Lab
http://www.scripps.edu/cb/schwartz/section1.htm
Eleni Tzima
http://www.med.unc.edu/physiolo/fac_tzima.htm
Prof. Masaaki
Sato
http://www.fmbe.coe.tohoku.ac.jp/studies/cfmo/msato-en.html
John A.
Frangos
http://www.ljbi.org/publicationsj.htm
Journals devoted to Shear Stress Research
Biophysical
journal
CAT.INIST
Nature
JCB
Cir Res
Circulation
Am J Physiol Heart Circ Physiol.
Webpage created by
GOPI KRISHNA KOLLURU
|